Magnesium Protects Tantalum, a Promising Material for Making Qubits
October 22, 2024
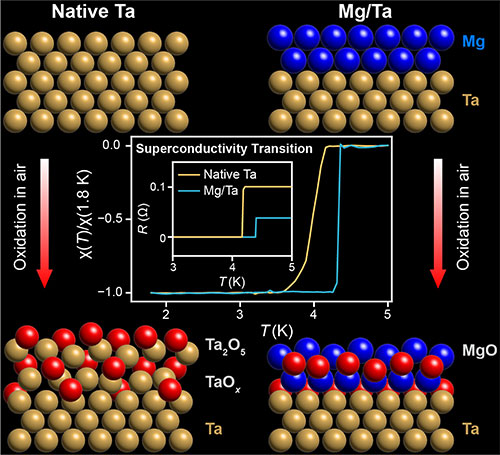
Magnesium capping protects tantalum, a promising superconducting qubit material, from oxidation
Scientific Achievement
C2QA researchers and collaborators have used CFN and NSLS II facilities to demonstrate that an ultrathin magnesium (Mg) capping layer significantly suppresses the formation of tantalum (Ta) oxide, enhancing the superconducting properties of Ta thin films.
Significance and Impact
By suppressing the formation of surface tantalum oxide, this novel Mg capping technique can remove a major dielectric loss channel in superconducting quantum materials, paving the way for high-performance quantum computing systems.
Research Details
Scaling up superconducting quantum circuits based on transmon qubits necessitates substantial enhancements in qubit coherence time. Over recent years, tantalum (Ta) has emerged as a promising candidate for transmon qubits, surpassing conventional counterparts in terms of coherence time. However, amorphous surface Ta oxide layer may introduce dielectric loss, ultimately placing a limit on the coherence time. In this study, we present a novel approach for suppressing the formation of tantalum oxide using an ultrathin magnesium (Mg) capping layer. Synchrotron-based X-ray photoelectron spectroscopy (XPS) studies demonstrate that Ta oxide is confined to an extremely thin region directly beneath the Mg/Ta interface. Additionally, we demonstrate that the superconducting properties of thin Ta films are improved following the Mg capping, exhibiting sharper and higher-temperature transitions to the superconductive and magnetically ordered state. Moreover, we establish an atomic-scale mechanistic understanding of the role of the capping layer in protecting Ta from oxidation based on computational modeling. This work provides valuable insights into the formation mechanism and functionality of surface tantalum oxide, as well as a new materials design principle with the potential to reduce dielectric loss in superconducting quantum materials. Ultimately, our findings pave the way for the realization of large-scale, high-performance quantum computing systems.
- Materials fabricated by magnetron sputtering (CFN)
- Low temperature transport measurements (CFN & CMPMSD) revealed higher superconducting transition temperature and sharper transition;
- Protective mechanism of the Mg layer were probed by synchrotron X-ray photoelectron spectroscopy (NSLS II/NIST) and electron microscopy (CFN & CMPMSD);
- Oxidation protection and oxygen gettering models are supported by first-principles calculation at PNNL.
Publication Reference
C. Zhou, J. Mun, J. Yao, A. Anbalagan, M. D. Hossain, R. A. McLellan, R. Li, K. Kisslinger, G. Li, X. Tong, A. R. Head, C. Weiland, S. L. Hulbert, A. L. Walter, Q. Li, Y. Zhu, P. V. Sushko, & M. Liu. “Ultrathin Magnesium-Based Coating as an Efficient Oxygen Barrier for Superconducting Circuit Materials.” Advanced Materials 36, 2310280 (2024).
Press release: Magnesium Protects Tantalum, a Promising Material for Making Qubits | BNL Newsroom
DOI: 10.1002/adma.202310280
OSTI: https://www.osti.gov/pages/biblio/2324577
Acknowledgment of Support
This project is supported by the U.S. Department of Energy (DOE), Office of Science (SC), National Quantum Information Science Research Centers (NQISRCs), Co-design Center for Quantum Advantage (C2QA) under contract number DE-SC0012704. The resources used in this research included the National Institute of Standards and Technology (NIST) operated beamlines Spectroscopy Soft and Tender Beamlines (SST-1 and SST-2), located at the National Synchrotron Light Source II (NSLS-II) and Materials Synthesis & Characterization and Electron Microscopy facilities of the Center for Functional Nanomaterials (CFN), DOE Office of Science Facilities at Brookhaven National Laboratory (BNL) under contract number DE-SC0012704. The authors acknowledged the use of facilities of Electron Microscopy and Nanostructure Group (Y.Z., J.M.) and Advanced Energy Materials Group (Q.L., J.Y.), Department of Condensed Matter Physics & Materials Science at BNL, primarily supported by the DOE, Office of Basic Energy Science (BES), Materials Science and Engineering Division, under contract number DE-SC0012704. Computational modeling at the Pacific Northwest National Laboratory (PNNL) was supported by C2QA (BES, PNNL FWP 76274). This research used resources of the National Energy Research Scientific Computing Center (NERSC), a DOE SC User Facility supported by the SC of the U.S. DOE under Contract No. DE-AC02-05CH11231 using NERSC Award No. BES-ERCAP0021800. We also thank Nathalie de Leon for useful discussions.
2024-22177 | INT/EXT | Newsroom