Visualizing and Understanding Reaction Paths in Lithium-ion Batteries
March 31, 2014
What Is The Scientific Achievement?
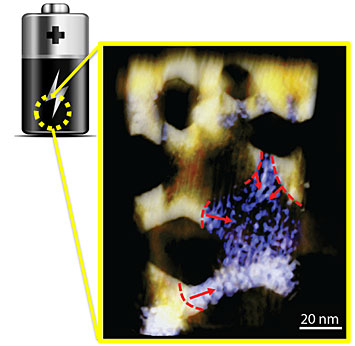
Heterogeneous phase conversion is dominant during the charging and discharging of nickel oxide (NiO) nanosheets within a lithium ion system. The contours and arrows in the image highlight the edges of the regions where the phase converted and the direction of the phase conversion that simultaneously propagated in a single NiO nanosheet.
The storage of energy for later use is of paramount importance for many of the technologies we use in our daily lives. In particular, lithium ion batteries find ubiquitous use in personal electronics and are promising candidates for applications in transportation. However, there remain many important practical problems with lithium ion batteries that have proven difficult to circumvent. These include a poor cycle lifetime and a low charging rate, as well as lingering safety concerns. Our inability to overcome these hurdles is due in large part to our lack of understanding of the reaction pathways that exist in a real lithium ion battery system. In this study, Brookhaven National Lab scientists led a team that consisted of four national laboratories, who worked together to combine several forefront characterization approaches, so as to provide a direct visualization of the reaction pathways in these systems. To evaluate and improve these lithium ion battery materials, they must be seen in action, while the battery is charging or discharging. This CFN-led collaboration developed a novel method that uses x-rays and electron microscopy to visualize the changes that occur during the charging of an electrode. The images reveal how a nickel oxide anode in a Li-ion battery is chemically transformed into metallic nickel during the charging process.
Why Does This Matter?
This novel experimental approach enabled the study of battery materials that are three-dimensionally submerged in a commercial liquid organic electrolyte, and yet remained amenable to imaging using state-of-the-art spatially-resolved fast electron spectroscopy and tomography. Further, these results shed light onto the origin of the inhomogeneous charge distribution in battery electrodes and will stimulate ways of improving battery performance. Also, the visualization method can be used to understand and assess many other electrode materials.
What Are The Details?
- CFN Capabilities: The Electron Microscopy Facility at CFN provided high resolution transmission electron microscopy for the study.
- The team discovered and reconstructed the three-dimensional nanostructures that evolve during the charging of lithium ion batteries. They found that the initiation of the reaction fronts is spatially distant, even within the same slab of material. This is in contrast to what was previously conjectured, namely that the reactions started uniformly from the surface of the material.
- In combination with synchrotron soft X-ray techniques, the dynamic changes to both the electrode surface structure and its interaction with the liquid electrolyte were characterized at complementary length scales, using electron microscopy, giving a comprehensive understanding of the evolving structure. In addition, the combination of X-ray and fast electron spectroscopy described here presents a new paradigm for correlated characterization of the evolution of chemistry in battery electrodes at complementary length scales.
Publication Reference
Phase Evolution for Conversion Reaction Electrodes in Lithium-ion Batteries
Feng Lin1,2, Dennis Nordlund3, Tsu-Chien Weng3, Ye Zhu4,5, Chunmei Ban6, Ryan M. Richards2, and Huolin L. Xin7
- Environmental Energy Technologies Division, Lawrence Berkeley National Laboratory, Berkeley, California 94720, USA.
- Department of Chemistry and Geochemistry, Materials Science Program, Colorado School of Mines, Golden, Colorado 80401, USA.
- Stanford Synchrotron Radiation Lightsource, SLAC National Accelerator Laboratory, Menlo Park, California 94025, USA.
- Monash Centre for Electron Microscopy, Monash University, Victoria 3800, Australia.
- Department of Materials Engineering, Monash University, Victoria 3800, Australia.
- Chemical and Materials Science Center, National Renewable Energy Laboratory, Golden, Colorado 80401, USA.
- Center for Functional Nanomaterials, Brookhaven National Laboratory, Upton, New York 11973, USA.
Nature Communications 5, 3358 (2014)
Acknowledgment of Support
Research carried out in part at the Center for Functional Nanomaterials, Brookhaven National Laboratory, which is supported by the U.S. Department of Energy, Office of Basic Energy Sciences, under Contract No. DE-AC02-98CH10886. This study also made use of NCEM facilities supported by the U.S. Department of Energy (DOE) under Contract No. DE-AC02-05CH11231, and Stanford Synchrotron Radiation Lightsource, a Directorate of SLAC National Accelerator Laboratory and an Office of Science User Facility operated for the U.S. Department of Energy Office of Science by Stanford University. Acknowledgement is also given to Dr. H. Zheng at LBNL her support and advice.
2014-4761 | INT/EXT | Newsroom