Platinum-Lead Core/Shell Nanoplates are High-Performance, Highly-Stable Fuel Cell Catalysts
February 13, 2017
What is the scientific achievement?
A team of scientists from CFN, Peking University, and Soochow University designed and characterized a new fuel cell catalyst — a platinum-lead core/shell structure, shaped as a nanoplate. The catalyst shape and chemical composition dramatically enhances the oxygen evolution reaction — important for fuel cell performance — while providing stability during operation. High-resolution studies show that tensile strain in the (110) plane of the platinum shell is key to high performance.
Why does this achievement matter?
The catalytic activity of new platinum-lead core/shell catalyst is nearly 10? higher than the 2020 DOE target for fuel cell performance.
What are the details?
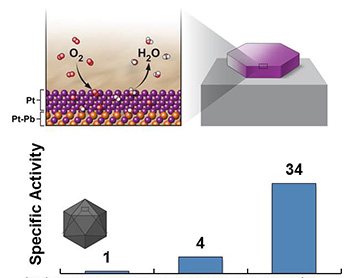
(Top) Schematic of the oxygen reduction reaction at the Pt/Pb nanoplate catalyst surface. (Bottom) The catalytic activity of the Pt/Pb nanoplate catalyst is 34 x higher than a standard Pt catalyst, and 4 x higher than a Pt/Pb catalyst with a different shape.
CFN Capabilities:
CFN Electron Microscopy Facility and staff modeling expertise were used to characterize the catalyst structure and internal strain.
Publication Reference
L. Bu, N. Zhang, S. Guo, X. Zhang, J. Li, J. Yao, T. Wu, G. Lu, J. –Y. Ma, D. Su and X. Huang Science, 354, 1610 (2016).
Press release (Brookhaven)
ScienceDaily
Phys.org
Sciencenewsline
greencarcongress
Esciencenews
Materials Science News-Elsevier
Acknowledgement of Support
This work was financially supported by the National Key Research and Development Program of China (No. 2016YFB0100201), the National Natural Science Foundation of China (21571135 and 51671003), the Ministry of Science and Technology (2016YFA0204100), the start-up fundings from Soochow University and Peking University, Young Thousand Talented Program and the Priority Academic Program Development of Jiangsu Higher Education Institutions (PAPD). Part of electron microscopy work was performed at the Center for Functional Nanomaterials, Brookhaven National Laboratory, which is supported by the U.S. Department of Energy (DOE), Office of Basic Energy Science, under Contract No. DE-SC0012704. The work at California State University Northridge was supported by the US Army Research Office via the MURI grant W911NF-11-1-0353.
2017-12212 | INT/EXT | Newsroom